Application of newly proposed hardening laws for structural steel rods
Author affiliations
DOI:
https://doi.org/10.15625/0866-7136/20106Keywords:
structure steel rods, hardening law, post-necking, finite element analysis, uniaxial tensile testAbstract
In civil engineering, distinct mechanical properties and behaviors of structural steel rods necessitate a novel approach to material modeling. This study extends the application of recently proposed strain-hardening laws, originally developed for automotive sheet metals, to several structural steel rods (CB240-T and CB300-T). Standard uniaxial tensile tests are conducted for each examined material to obtain experimental stress-strain data. Various curve fitting methods are then employed to refine the parameters of the strain-hardening laws, enabling accurate representation of the steel rods mechanical behavior. Subsequently, these laws are implemented in Abaqus software for numerical simulation of uniaxial tensile tests, facilitating the analyses of material response under uniaxial tensile loading condition. Compared to the measured data, the predicted force-displacement curves are in good agreement with the measurements until the tail of the curves. The comparisons verify the ability and potential of the examined hardening law for studying the post-necking behavior of structural steels. The outcomes provide a framework for more precise characterization of structural steel materials.
Downloads
References
M. B. Jabłońska, K. Kowalczyk, M. Tkocz, R. Chulist, K. Rodak, I. Bednarczyk, and A. Cichański. The effect of severe plastic deformation on the IF steel properties, evolution of structure and crystallographic texture after dual rolls equal channel extrusion deformation. Archives of Civil and Mechanical Engineering, 21, (2021).
A. A. Shah and Y. Ribakov. Recent trends in steel fibered high-strength concrete. Materials & Design, 32, (2011), pp. 4122–4151.
S. L. Chan. Non-linear behavior and design of steel structures. Journal of Constructional Steel Research, 57, (2001), pp. 1217–1231.
Z.-J. Zhang, B.-S. Chen, R. Bai, and Y.-P. Liu. Non-linear behavior and design of steel structures: Review and outlook. Buildings, 13, (2023).
W. Ramberg and W. R. Osgood. Description of stress-strain curves by three parameters. Technical report, National Advisory Committee for Aeronautics, (1943).
J. H. Hollomon. Tensile deformation. Transactions of AIME, 162, (1945), pp. 268–290.
H.W. Swift. Plastic instability under plane stress. Journal of the Mechanics and Physics of Solids, 1, (1952), pp. 1–18.
D. C. Ludwigson. Modified stress-strain relation for FCC metals and alloys. Metallurgical Transactions, 2, (1971), pp. 2825–2828.
N. Y. Golovina. The nonlinear stress-strain curve model as a solution of the fourth order differential equation. International Journal of Pressure Vessels and Piping, 189, (2021).
A. Lavakumar, S. S. Sarangi, V. Chilla, D. Narsimhachary, and R. K. Ray. A “new” empirical equation to describe the strain hardening behavior of steels and other metallic materials. Materials Science and Engineering: A, 802, (2021).
T. Li, J. Zheng, and Z. Chen. Description of full-range strain hardening behavior of steels. SpringerPlus, 5, (2016).
Q. T. Pham and Y. S. Kim. Identification of the plastic deformation characteristics of AL5052-sheet based on the non-associated flow rule. Metals and Materials International, 23, (2017), pp. 254–263.
Q. T. Pham and Y.-S. Kim. Evaluation on flexibility of phenomenological hardening law for automotive sheet metals. Metals, 12, (2022).
Q. T. Pham, H. S. Le, A. T. Nguyen, X. Xiao, Y.-S. Kim, V. D. Nguyen, H. S. Tran, and X. Van Tran. A machine learning–based methodology for identification of the plastic flow in aluminum sheets during incremental sheet forming processes. The International Journal of Advanced Manufacturing Technology, 120, (2022), pp. 3559–3584.
Q. T. Pham, T. Nguyen-Thoi, J. Ha, and Y.-S. Kim. Hybrid fitting-numerical method for determining strain-hardening behavior of sheet metals. Mechanics of Materials, 161, (2021).
J. Vetrone, J. E. Obregon, E. J. Indacochea, and D. Ozevin. The characterization of deformation stage of metals using acoustic emission combined with nonlinear ultrasonics. Measurement, 178, (2021).
TCVN 1651-1:2018. Steel for the reinforcement of concrete - Part 1. Vietnamese National Standard, Ministry of Construction.
Dassault Systèmes®. Abaqus analysis user’s guide, (2023).
M. Kõrgesaar. The effect of low stress triaxialities and deformation paths on ductile fracture simulations of large shell structures. Marine Structures, 63, (2019), pp. 45–64.
S. Marth, H.-Å. Häggblad, M. Oldenburg, and R. Östlund. Post necking characterisation for sheet metal materials using full field measurement. Journal of Materials Processing Technology, 238, (2016), pp. 315–324.
S.-J. Park, B. C. Cerik, and J. Choung. Comparative study on ductile fracture prediction of high-tensile strength marine structural steels. Ships and Offshore Structures, 15, (2020), pp. S208–S219.
Z. Yao and W. Wang. Full-range strain-hardening behavior of structural steels: Experimental identification and numerical simulation. Journal of Constructional Steel Research, 194, (2022).
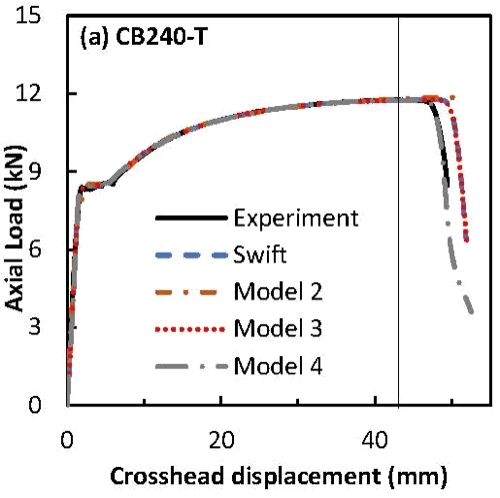
Downloads
Published
License

This work is licensed under a Creative Commons Attribution-ShareAlike 4.0 International License.