Vibroacoustic behavior of a rectangular composite plate in thermal environment
Author affiliations
DOI:
https://doi.org/10.15625/0866-7136/17936Keywords:
vibroacoustic behavior, laminated composite plate, sound transmission loss, thermal loadsAbstract
This paper is a study of the vibroacoustic behavior of orthotropic laminated composite rectangular plate under a sound wave excitation in thermal environments. An improved analytical procedure has been developed that allows for an efficient solution of the finite composite plate sound transmission problem. A symmetrically orthotropic laminated composite plate is considered. The plate is modeled with classic thin-plate theory and is assumed to be clamped on all four sides. The incident acoustic pressure is modeled as a harmonic plane wave impinging on the plate at an arbitrary angle. The sound transmission loss is calculated from the ratio of incident to transmitted acoustic powers.
Downloads
References
S. H. Ko. Reduction of structure-borne noise using an air-voided elastomer. The Journal of the Acoustical Society of America, 101, (1997), pp. 3306–3312.
A. Tadeu and J. Antonio. Acoustic insulation of single panel walls provided by analytical expressions versus the mass law. Journal of Sound and Vibration, 257, (2002), pp. 457–475.
A. Tadeu, J. Antonio, and N. Simoes. Acoustic insertion loss provided by single and double steel panels separating an air from a water medium. Acta Acoustica/Acustica, 89, (3), (2003), pp. 391–405.
H.-J. Lin, C.-N. Wang, and Y.-M. Kuo. Sound transmission loss across specially orthotropic laminates. Applied Acoustics, 68, (2007), pp. 1177–1191.
Y.-M. Kuo, H.-J. Lin, and C.-N. Wang. Sound transmission across orthotropic laminates with a 3D model. Applied Acoustics, 69, (2008), pp. 951–959.
T. I. Thinh and P. N. Thanh. Vibroacoustic response of a finite clamped laminated composite plate. In Advances in Engineering Research and Application, pp. 589–600. Springer International Publishing, (2018).
B. Pan, D. Wu, and Y. Xia. High-temperature deformation field measurement by combining transient aerodynamic heating simulation system and reliability-guided digital image correlation. Optics and Lasers in Engineering, 48, (2010), pp. 841–848.
B. Budiansky and J. Mayers. Influence of aerodynamic heating on the effective torsional stiffness of thin wings. Journal of the Aeronautical Sciences, 23, (1956), pp. 1081–1093.
H. V. Tung. Nonlinear thermomechanical response of pressure-loaded doubly curved functionally graded material sandwich panels in thermal environments including tangential edge constraints. Journal of Sandwich Structures & Materials, 20, (2017), pp. 974–1008.
Y. Frostig. Classical and high-order computational models in the analysis of modern sandwich panels. Composites Part B: Engineering, 34, (2003), pp. 83–100.
Z. Sun, S. Shi, X. Guo, X. Hu, and H. Chen. On compressive properties of composite sandwich structures with grid reinforced honeycomb core. Composites Part B: Engineering, 94, (2016), pp. 245–252.
Z. Wang, J. Liu, Z. Lu, and D. Hui. Mechanical behavior of composited structure filled with tandem honeycombs. Composites Part B: Engineering, 114, (2017), pp. 128–138.
U. Caliskan and M. K. Apalak. Low velocity bending impact behavior of foam core sandwich beams: Experimental. Composites Part B: Engineering, 112, (2017), pp. 158–175.
M. Mohammadimehr and S. Shahedi. High-order buckling and free vibration analysis of two types sandwich beam including AL or PVC-foam flexible core and CNTs reinforced nanocomposite face sheets using GDQM. Composites Part B: Engineering, 108, (2017), pp. 91–107.
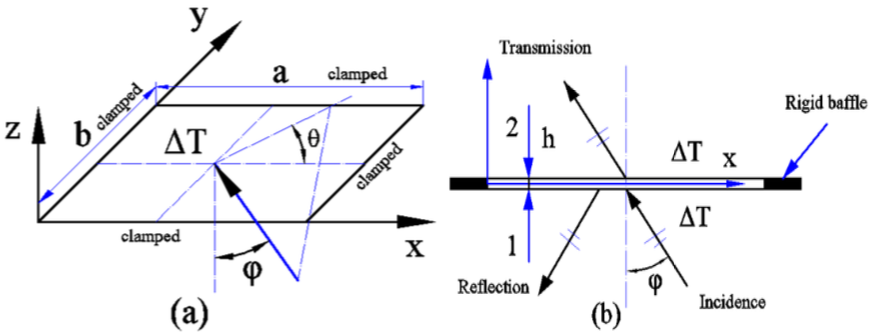
Downloads
Published
How to Cite
License

This work is licensed under a Creative Commons Attribution-ShareAlike 4.0 International License.