Experimental and numerical evaluation of thermodynamic effect on NACA0015 hydrofoil cavitation in hot water
Author affiliations
DOI:
https://doi.org/10.15625/0866-7136/15764Keywords:
cavitation, computational fluid dynamics, thermodynamic, NACA0015Abstract
In this study, the cavitation in hot water, which implies tight interaction of thermodynamic effect, phase change phenomena, and flow behavior, was studied by a combination of experiment and numerical simulation. The experiment in water up to 90°C was performed in the high temperature and high-pressure water tunnel with NACA0015 as a cavitator. The temperature inside the cavity was measured using the high-accuracy thermistor probe. According to the result, the temperature depression in the cavity was increased proportionally with the increase of freestream temperature. The inverse thermodynamic effect was observed with the increase of cavity length when temperature increased. The maximum temperature depression of about 0.41°C was measured in the water at around 90°C. The temperature drop was reasonably captured in simulation by coupling our simplified thermodynamic model with our cavitation model and governing equations. The tendency of temperature depression in the cavity agreed well with experimental data under different flow conditions.
Downloads
References
M. Petkovšek and M. Dular. IR measurements of the thermodynamic effects in cavitating flow. International Journal of Heat and Fluid Flow, 44, (2013), pp. 756–763. DOI: https://doi.org/10.1016/j.ijheatfluidflow.2013.10.005
M. Petkovšek and M. Dular. Observing the thermodynamic effects in cavitating flow by IR thermography. Experimental Thermal and Fluid Science, 88, (2017), pp. 450–460. DOI: https://doi.org/10.1016/j.expthermflusci.2017.07.001
Y. Yamaguchi and Y. Iga. Thermodynamic effect on cavitation in high temperature water. In Fluids Engineering Division Summer Meeting, American Society of Mechanical Engineers, (2014). DOI: https://doi.org/10.1115/FEDSM2014-21433
A. Cervone, C. Bramanti, E. Rapposelli, and L. d’Agostino. Thermal Cavitation Experiments on a NACA 0015 Hydrofoil. Journal of Fluids Engineering, 128, (2005), pp. 326–331. DOI: https://doi.org/10.1115/1.2169808
S.-I. Tsuda, N. Tani, and N. Yamanishi. Development and Validation of a Reduced Critical Radius Model for Cryogenic Cavitation. Journal of Fluids Engineering, 134, (2012). DOI: https://doi.org/10.1115/1.4006469
A. Hosangadi and V. Ahuja. Numerical Study of Cavitation in Cryogenic Fluids. Journal of Fluids Engineering, 127, (2005), pp. 267–281. DOI: https://doi.org/10.1115/1.1883238
Y. Utturkar, J. Wu, G. Wang, and W. Shyy. Recent progress in modeling of cryogenic cavitation for liquid rocket propulsion. Progress in Aerospace Sciences, 41, (2005), pp. 558–608. DOI: https://doi.org/10.1016/j.paerosci.2005.10.002
C. C. Tseng and W. Shyy. Turbulence Modeling for Isothermal and Cryogenic Cavitation. In 47th AIAA Aerospace Sciences Meeting including The New Horizons Forum and Aerospace Exposition, American Institute of Aeronautics and Astronautics, (2009), DOI: https://doi.org/10.2514/6.2009-1215
A. D. Le, J. Okajima, and Y. Iga. Modification of Energy Equation for Homogeneous Cavitation Simulation With Thermodynamic Effect. Journal of Fluids Engineering, 141, (2019). DOI: https://doi.org/10.1115/1.4042257
A. D. Le, J. Okajima, and Y. Iga. Numerical simulation study of cavitation in liquefied hydrogen. Cryogenics, 101, (2019), pp. 29–35. DOI: https://doi.org/10.1016/j.cryogenics.2019.04.010
H.-T. Chen and R. Collins. Shock wave propagation past an ocean surface. Journal of Computational Physics, 7, (1971), pp. 89–101. DOI: https://doi.org/10.1016/0021-9991(71)90051-9
Y. Iga, M. Nohmi, A. Goto, B. R. Shin, and T. Ikohagi. Numerical Study of Sheet Cavitation Breakoff Phenomenon on a Cascade Hydrofoil. Journal of Fluids Engineering, 125, (2003), pp. 643–651. DOI: https://doi.org/10.1115/1.1596239
Y. Iga, M. Nohml, A. Goto, and T. Ikohagi. Numerical Analysis of Cavitation Instabilities Arising in the Three-Blade Cascade. Journal of Fluids Engineering, 126, (2004), pp. 419–429. DOI: https://doi.org/10.1115/1.1760539
D. C. Wilcox. Turbulence modeling for CFD. DCW industries La Canada, CA, (1994).
N. Ochiai, Y. Iga, M. Nohmi, and T. Ikohagi. Numerical Prediction of Cavitation Erosion Intensity in Cavitating Flows around a Clark Y 11.7% Hydrofoil. Journal of Fluid Science and Technology, 5, (3), (2010), pp. 416–431. DOI: https://doi.org/10.1299/jfst.5.416
A. K. Singhal, M. M. Athavale, H. Li, and Y. Jiang. Mathematical Basis and Validation of the Full Cavitation Model. Journal of Fluids Engineering, 124, (2002), pp. 617–624. DOI: https://doi.org/10.1115/1.1486223
R. Maccormack. The effect of viscosity in hypervelocity impact cratering. In 4th Aerodynamic Testing Conference, American Institute of Aeronautics and Astronautics, (1969). DOI: https://doi.org/10.2514/6.1969-354
H. C. Yee. Upwind and Symmetric Shock - Capturing Schemes. NASA Technical Memorandum 89464, (1987).
F. Menter and T. Esch. Elements of industrial heat transfer predictions. In 16th Brazilian Congress of Mechanical Engineering (COBEM), Uberlandia, Brazil, (2001).
C. Brennen. The Dynamic Behavior and Compliance of a Stream of Cavitating Bubbles. Journal of Fluids Engineering, 95, (1973), pp. 533–541. DOI: https://doi.org/10.1115/1.3447067
S.Watanabe, T. Hidaka, H. Horiguchi, A. Furukawa, and Y. Tsujimoto. Steady Analysis of the Thermodynamic Effect of Partial Cavitation Using the Singularity Method. Journal of Fluids Engineering, 129, (2006), pp. 121–127. DOI: https://doi.org/10.1115/1.2409333
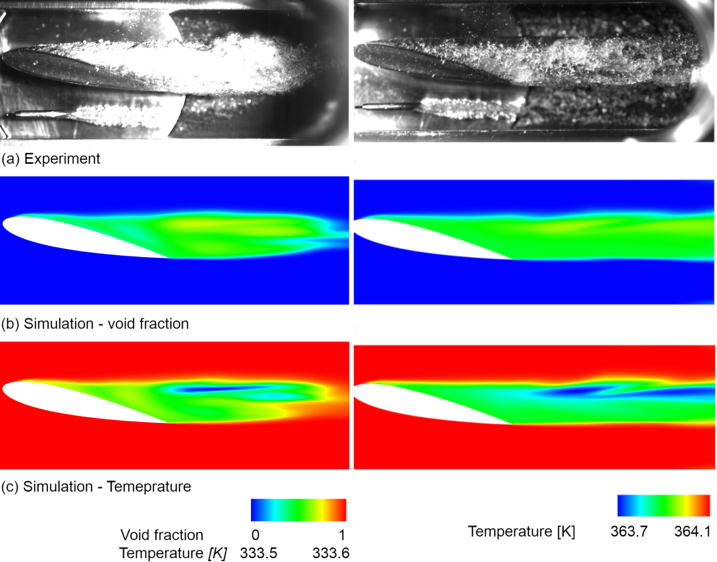
Downloads
Published
Issue
Section
License

This work is licensed under a Creative Commons Attribution-ShareAlike 4.0 International License.
Funding data
-
National Foundation for Science and Technology Development
Grant numbers 107.03-2020.22